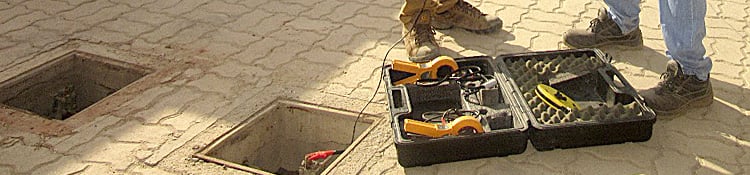
Electrical safety testing is essential to ensure safe operating standards for any product that uses electricity. Various governments and agencies have developed stringent requirements for electrical products that are sold world-wide. Several tests are conducted to check the safety of products. One among that is earth test.
Potentially the most dangerous appliances are Class I appliances (earthed appliances), example: microwave ovens/bench grinders and the like, but also in this category are extension leads. Class I appliances are designed to have a connection to the ground via an earth conductor. This may, or may not, be a suitable low resistance path for electric current to protect personnel and equipment. If this conductor is damaged anywhere then the consequences can be virulent.
Why is Earth Ground Testing Needed?
The measurement of ground resistance for an earth electrode system should be done when the electrode is first installed, and then at periodic intervals thereafter. This ensures that the resistance-to-ground does not increase over time. The International Electrical Testing Association specifies ground electrode testing every three years for a system in good condition with average up-time requirements.
Poor grounding not only increases the risk of equipment failure; it is dangerous too. Facilities need to have adequately grounded electrical systems so in the event of a lightning strike, or utility overvoltage, current will find a safe path to earth. Although the ground system when initially installed had low earth ground resistance values, the resistance of the grounding system can increase if the ground rods are eaten away by corrosive soils with high moisture content, high salt content, and high temperatures.
If our technician finds an increase in resistance of more than 20 percent, we will investigate the source of the problem and make the correction to the grounding system to lower the resistance.
Factors That Can Change Your Minimum Earth Resistance
- A plant or other electrical facility can expand in size. Also, new plants continue to be built larger and larger. Such changes create different needs in the earth electrode. What was formerly a suitably low earth resistance can become an obsolete “standard.”
- As facilities add more modern sensitive computer-controlled equipment, the problems of electrical noise is magnified. Noise that would not effect cruder, older equipment can cause daily problems with new equipment.
- As more non-metallic pipes and conduits are installed underground, such installations become less and less dependable as effective, low-resistance ground connections.
- In many locations, the water table is gradually falling. In a year or so, earth electrode systems that formerly were effective may end up in dry earth of high resistance.
These factors emphasize the importance of a continuous, periodic program of earth-resistance testing. It is not enough to check the earth resistance only at the time of installation.
Factors Influencing Requirements for a Good Grounding System
- Limiting to definite values the voltage to earth of the entire electrical system. Use of a suitable grounding system can do this by maintaining some point in the circuit at earth potential. Such a grounding system provides these advantages:
- Limits voltage to which the system-to-ground insulation is subjected, thereby more definitely fixing the insulation rating.
- Limits the system-to-ground or system-to-frame voltage to values safe for personnel.
- Provides a relatively stable system with a minimum of transient over-voltage.
- Permits any system fault to ground to be quickly isolated.
- Proper grounding of metallic enclosures and support structures that are part of the electrical system and may be contacted by personnel. Also to be included are portable electrically operated devices. Consider that only a small amount of electric current — as little as 01 A for one second — can be fatal! An even smaller amount can cause you to lose muscular control. These low currents can occur in your body at voltages as low as 100 V, if your skin is moist.
- Protection against static electricity from friction. Along with this are the attendant hazards of shock, fire and explosion. Moving objects that may be inherent insulators – such as paper, textiles, conveyor belts or power belts and rubberised fabrics – can develop surprisingly high charges unless properly grounded.
- Protection against direct lightning strokes. Elevated structures, such as stacks, the building proper, and water tanks may require lightning rods connected into the grounding system.
- Protection against induced lightning voltages. This is particularly a factor if aerial power distribution and communications circuits are involved. Lightning arresters may be required in strategic locations throughout the plant.
- Providing good grounds for electric process control and communication circuits. With the increased use of industrial control instruments, computers, and communications equipment, accessibility of low resistance ground connections in many plant locations — in office and production areas — must be considered.
Resistance to earth can vary with changes in climate and temperature. Such changes can be considerable. An earth electrode that was good (low-resistance) when installed may not stay that way; to be sure, you must check it periodically. We cannot tell you what your maximum earth resistance should be. For specific systems in definite locations, specifications are often set. Some call for 5 Ω maximum; others accept no more than 3 Ω. In certain cases, resistances as low as a small fraction of an ohm are required.
Nature of an Earth Electrode
Nature of an Earth Electrode Resistance to current through an earth electrode actually has three components:
- Resistance of the electrode itself and connections to it.
- Contact resistance between the electrode and the soil adjacent to it.
- Resistance of the surrounding earth.
Electrode Resistance: Rods, pipes, masses of metal, structures, and other devices are commonly used for earth connections. These are usually of sufficient size or cross-section that their resistance is a negligible part of the total resistance.
Electrode-Earth Contact Resistance: This is much less than you might think. If the electrode is free from paint or grease, and the earth is packed firmly, contact resistance is negligible. Rust on an iron electrode has little or no effect but if an iron pipe has rusted through, the part below the break is not effective as a part of the earth electrode
Resistance of Surrounding Earth: An electrode driven into earth of uniform resistivity radiates current in all directions. Think of the electrode as being surrounded by shells of earth, all of equal thickness. The earth shell nearest the electrode naturally has the smallest surface area and so offers the greatest resistance
Principles Involved in Earth Resistance Testing
The resistance to earth of any system of electrodes theoretically can be calculated from formulas based upon the general resistance formula:
R = ρ LA
Where ρ is the resistivity of the earth in ohm-cm, L is the length of the conducting path, and A is the cross-sectional area of the path. All such formulas can be simplified a little by basing them on the assumption that the earth’s resistivity is uniform throughout the entire soil volume under consideration.
There are five basic test methods as noted below
Soil Resistivity Testing:
Wenner four-pole equal method [19] has been considered in measuring the soil resistivity. The correct design of an earthing system is dependent upon detailed knowledge of the local ground resistivity. This is measured as a function of depth at a series of locations around the site, using an expanding four electrode Wenner array (BS EN 50522). The procedure is known as soil resistivity or earth resistance testing. Correct measurement is particularly important in areas of high resistivity ground, where electrical currents are not able to dissipate. In these conditions obtaining an earth can be problematic, and information on ground resistivity is required to much greater depths for the successful installation of an earthing system.
Fall-of-Potential Method:
With a four-terminal tester, P1 and C1 terminals on the instrument are connected to the earth electrode under test. With a three-terminal instrument, connect X to the earth electrode. Although four terminals are necessary for resistivity measurements, the use of either three of four terminals is largely optional for testing the resistance of an installed electrode. The use of three terminals is more convenient because it requires one lead to be connected. The trade-off is that the resistance of this common lead is included in the measurement. Normally, this effect can be minimized by keeping the lead short, to accommodate simple test requirements. The small additional resistance thus introduced is negligible. When performing more complex tests or meeting stringent requirements, however, it may be better to use all four terminals by a lead from the P1 terminal to the test electrode (connecting it inside the lead from C1). This is a true four wire test configuration which eliminates all lead resistance from the measurement.
The added accuracy may prove significant when meeting very low resistance specifications or using test methods that necessitate an extra digit of measurement in order to meet the mathematical requirements. The decision is optional, based on the operator’s testing goals and the method used. The driven reference rod C should be placed as far from the earth electrode as practical; this distance may be limited by the length of extension wire available, or the geography of the surroundings. Leads should be separated and “snaked,” not run close and parallel to each other, to eliminate mutual inductance. Potential-reference rod P is then driven in at a number of points roughly on a straight line between the earth electrode and C. Resistance readings are logged for each of the points.
Dead Earth Method:
When using a four-terminal instrument, P1 and C1 terminals connect to the earth electrode under test; P2 and C2 terminals connect to an all-metallic water-pipe system. With a three-terminal instrument, connect X to the earth electrode, P and C to the pipe system. If the water system is extensive (covering a large area), its resistance should only be a fraction of an ohm. You can then take the instrument reading as being the resistance of the electrode under test. The dead earth method is the simplest way to make an earth resistance test. With this method, resistance of two electrodes in series is measured — the driven rod and the water system. But there are three important limitations:
- The waterpipe system must be extensive enough to have a negligible resistance.
- The waterpipe system must be metallic throughout, without any insulating couplings or flanges.
- The earth electrode under test must be far enough away from the water-pipe system to be outside its sphere of influence. In some locations, your earth electrode may be so close to the water-pipe system that you cannot separate the two by the required distance for measurement by the two-terminal method.
Under these circumstances, if conditions 1 and 2 above are met, you can connect to the water-pipe system and obtain a suitable earth electrode. As a precaution against any possible future changes in the resistance of the water-pipe system, however, you should also install an earth electrode.
Clamp-on Method:
Fall-of-potential testing, and its modifications, is the only ground testing method that conforms to IEEE 81. It is extremely reliable, highly accurate and can be used to test any size ground system. Additionally, the operator has complete control of the test set-up and can check or proof his/her results by testing at different probe spacing. Unfortunately, the Fall of Potential method also comes with drawbacks:
- It is extremely time consuming and labour intensive.
- Individual ground electrodes must be disconnected from the system to be measured.
The clamp-on ground testing method, although it does not conform to IEEE 81, does provide the operator with the ability to make effective measurements under the right conditions. The clamp-on methodology is based on Ohm’s Law (R=V/I). A known voltage is applied to a complete circuit and the resulting current flow is measured. The resistance of the circuit can then be calculated. The clamp-on ground tester applies the signal and measures the current without a direct electrical connection. The clamp includes a transmit coil that applies the voltage and a receive coil that measures the current.
Selective Measurement Testing:
Selective testing is very similar to the Fall-of-Potential testing, providing all the same measurements, but in a much safer and easier way. This is because with Selective testing, the earth electrode of interest does not need to be disconnected from its connection to the site! The technician does not have to endanger himself by disconnecting ground, nor endanger other personnel or electrical equipment inside a no grounded structure.
How to Improve Earth Resistance
When you find that your earth electrode resistance is not low enough, there are several ways you can improve it:
- Lengthen the earth electrode in the earth.
- Use multiple rods.
- Treat the soil.
Effect of Rod Size:
As you might suspect, driving a longer rod deeper into the earth, materially decreases its resistance. In general, doubling the rod length reduces resistance by about 40 percent.
Use of Multiple Rods:
Two well-spaced rods driven into the earth provide parallel paths. They are, in effect, two resistances in parallel. The rule for two resistances in parallel does not apply exactly; that is, the resultant resistance is not one-half the individual rod resistances (assuming they are of the same size and depth).
Treatment of the Soil:
Chemical treatment of soil is a good way to improve earth electrode resistance when you cannot drive deeper ground rods because of hard underlying rock, for example. It is beyond the scope of this manual to recommend the best treatment chemicals for all situations. You have to consider the possible corrosive effect on the electrode as well as EPA and local environmental regulations. Magnesium sulfate, copper sulfate, and ordinary rock salt are suitable non-corrosive materials. Magnesium sulfate is the least corrosive, but rock salt is cheaper and does the job if applied in a trench dug around the electrode. It should be noted that soluble sulfates attack concrete, and should be kept away from building foundations. Another popular approach is to backfill around the electrode with a specialized conductive concrete. A number of these products, like bentonite, are available on the market.
Effect of Temperature on Earth Resistivity
Not much information has been collected on the effects of temperature. Two facts lead to the logical conclusion that an increase in temperature will decrease resistivity:
- Water present in soil mostly determines the resistivity
- An increase in temperature markedly decreases the resistivity of water.
- The resistivity continues to increase as temperatures go below freezing.
Grounding testers are indispensable troubleshooting tools to help you maintain uptime. It is recommended that all grounds and ground connections be checked at least annually as a part of your normal predictive maintenance plan. Should an increase in resistance of more than 20% be measured during these periodic checks, the technician should investigate the source of the problem and make the correction to lower the resistance by replacing or adding ground rods to the ground system.